What Is Battery C Rating?
The battery C rating can be defined as the measure at which a battery is discharged relative to the maximum capacity of the batteries.
A battery’s charge and discharge rates are controlled by battery C rating. In other terms, it is the governing measure of at what current the intended batteries is charged or discharged and how quickly that occurs.
The capacity of a battery is generally rated and labeled at 3C rate(3C current), this means a fully charged battery with a capacity of 100Ah should be able to provide 3*100Amps current for one third hours, That same 100Ah battery being discharged at a C-rate of 1C will provide 100Amps for one hours, and if discharged at 0.5C rate it provide 50Amps for 2 hours.
The C rate is very important to know as with the majority of batteries the available stored energy depends on the speed of the charge and discharge currents.
Why The C Rating Are Different Between Different Battery?
1C means 1 hour discharge time.
2C means 1/2 hour discharge time.
0.5C means 2 hour discharge time.
In many applications, the battery rate is very important. For example, we want the car to be fully charged within half an hour, instead of waiting for 2 hours, or even 8 hours. What is cause influence to the battery C rating?
There are two limitations to how fast a battery can be charged-thermal heating and mass transfer limitations.
Thermal heating occurs because the internal resistance of the battery generates excessive heat, which must be dissipated to the environment.
When charging occurs at very high currents, the heat generated within the battery cannot be removed fast enough, and the temperature quickly rises.
Mass transfer of Li+ ions during fast charge results in diffusion limiting current even if the electrodes are made of nanoparticles with high surface area. While the high surface area allows sufficient rate of lithiation or de-lithiantion, the Li+ diffusion through the cross-sectional area of the electrolyte within the separator is limited. It is quite possible to fast- charge for a limited time restricted to the Li-ions already presented in the electrolyte withing the electrode. This unssteady state diffusion can last until the Li+ ions are depleted and their supply is limited by the cross-sectional area of the battery.
This mass transfer limitation occurs because the transference number of Li+ is smaller than 1. While Li+ions carry a fraction of the current in the electrolyte, they carry 100% of the current at the electrode; thus depletion of Li+ occurs near the anode, resulting in diffusion limiting current. Any attempt to surpass the limiting current results in solvent decomposition, heating and deterioration of the battery.
So different material battery will have different rate, the typical NCM lithium battery C rating is 1C, and maxium C rate can reach 10C about 18650 battery. the typical LiFePO4 lithium battery C rating is 1C, and the maxium C rate can reach 3C about LiFePO4 prismatic battery.
Battery C Rating Chart
Below chart shows the different battery C rating and their discharge time.When we caculate them, the battery C rating should use same caculation as the same energy.
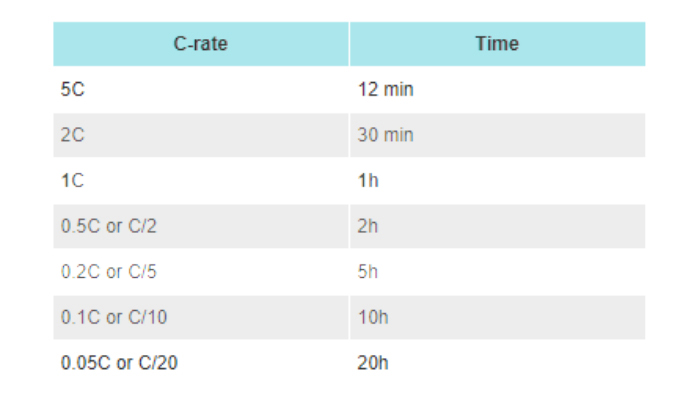
Battery C Rating Chart
For most of lithium battery, here is the picture to show the discharge curve in different C rate.
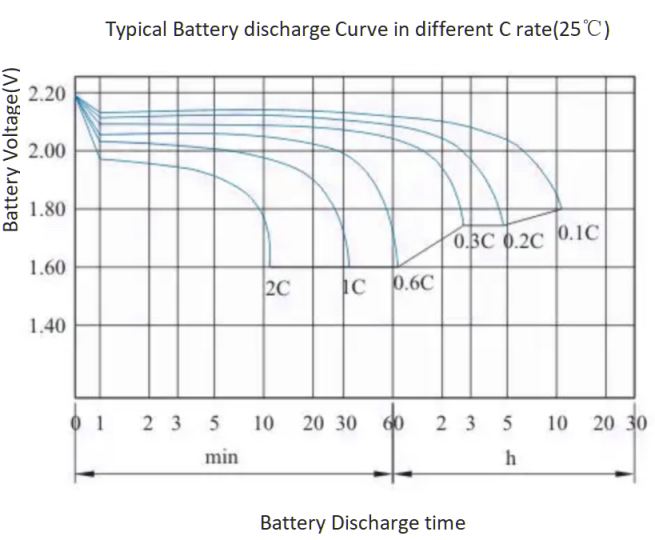
Battery Discharge Curve In Different Battery C Rating
For most lead-acid batteries, we should know that even for the same battery, the battery capacity at different battery C rating is different. To get a reasonably good capacity reading, lead acid batteries manufacturers typically rate lead-acid batteries at 20 hours(A very low 0.05C).
How To Calculate The C Rating For The Battery?
A battery’s C rating is defined by the time of charge and discharge.
C-rate is an important information or data for any battery, if a rechargeable battery can be discharged at that C rating, a 100Ah battery will provide about 100A, then the battery has a discharge rate of 1C. If the battery can only provide a maximum discharge current of about 50A, then the discharge rate of the battery is 50A/100Ah=0.5C.
C-rate (C) = charge or discharge current in amperes (A) / rated capacity of the battery(Ah)
Therefore, calculating the C rating is important for any battery user and can be used to derive output current, power and energy by:
Cr = I/Er
Er = Rated energy stored in Ah
I = Charge/discharge current in A
Cr = C rate of the battery
t = Charge/discharge duration
Calculate charge and discharge time
t = Er / I
100Ah Lithium Battery C Rate Example
For same 100Ah lithium battery,
1C means 100Ah*1C=100A discharge current available.
1C means 100Ah/100A=1 hours discharge time Capable.
It means the battery can be use for 60minute (1h) with load current of 100A.
2C means 100Ah*2C=200A discharge current available.
2C means 200Ah/100A=0.5 hours discharge time Capable.
It means the battery can be use for 30minute (0.5h) with load current of 200A.
0.5C means 100Ah*0.5C=50A discharge current available.
0.5C means 100Ah/50A=2 hours discharge time Capable.
It means the battery can be use for 120minute (2h) with load current of 50A.
Sometimes analyzer capacity readings are given as a percentage of the nominal rating. For example, if a 1000mAh battery can supply this current for about 60 minutes, read 100%. However, if the battery lasts only half an hour before the cut-off point, the displayed value is 50%. Sometimes a brand new battery can provide more than 100% capacity. The battery can be discharged using an analyzer which allows you to set your favorite C rate. If the battery is discharged at a lower discharge rate it will show a higher reading and vice versa. However, you should be aware of differences in battery analyzer capacity readings for different C rates, which are related to the internal resistance of the battery.
What Are The Effects Of C Rating On Lithium-ion Batteries?
After we caculated above, we know more higher the C rating on a battery, the faster the energy can escape the batteries to power the application. The C rating on any battery depends on its application. Because some electronics require large amounts of power supply thus need batteries with high C ratings, For example, the motorcycle starter, you only needs needs a few seconds to power the motors quickly. But for some application, the discharge time only need need low C rating, Such as the soalr light, you want them to power for whole night or several nights.
What Is The C Rating Of My Battery?
You’ll usually find the battery’s C-rate on the battery’s label and on the battery’s data sheet. Different battery chemistries sometimes show different battery C rates.
Generally speaking,
Lithium iron phosphate batteries typically have a discharge rate of 1C
NCM batteries typically have a discharge rate of 3C
Lead-acid batteries are generally rated for a very low discharge rate, typically 0.05C, or 20 hour rate.
If you cannot find the battery C rating on the label or datasheet, we recommend contacting the battery manufacturer directly.
In Conclusion
The C-rate is a unit used to identify a current value/discharge time of a lithium-ion battery under different conditions. Since you have had a clear view of what the C rating is , and what it stands for in a battery, you will need to include it in your next selection for batteries to get the best out of what you settle for.